A proton – in conditions here on Earth – consists of three electron-positron particles (analogous to three quarks). Two opposing electron-positron (e-+/e+- and e+-/e-+) particles are entangled with each other, each composing an opposing pole of the proton (along its axis of spin). The other electron-positron particle is “trapped” within the proton at its system center, where the 3-D electric energy is at its highest energy density, and where the gravitational energy gradient is the strongest. The proton has a radius analogous to the Schwarzchild radius of large bodies of mass.
Formation of Hydrogen atoms ….
The vast majority of protons exist in nature as nuclei of Hydrogen atoms – not as ‘charged’ particles. Hydrogen atoms may be formed under relatively extreme temperature and pressure at the core of stars. In such an extreme environment, a Hydrogen atom may first start out as a proton with four constituent particles within its boundary. The four constituent particles consist of two pairs of entangled e-+/ e+- particles (designated as an e-+/e+- entangled pair …. the -+ or +- show that the particle possesses alternating e-m directionality [inward to system center or outward from system center] with every e-m interaction). The two pairs of entangled e-+ and e+- particles may also be entangled with each other.
This predecessor to the Hydrogen atom then consists of a ‘naked’ proton with four constituent e-+ and e+- particles that may interact very closely with other identical ‘naked’ protons. As the ‘naked’ protons (each with four constituent e-+/e+- particles) move away from the core of a star into regions of lower pressure and temperature, the proton structure becomes less stable due to an excess number of e-+/e+- particles occupying available nuclear energy levels in this environment of lower pressure and temperature.
It is likely that these Hydrogen ‘atoms’ (i.e., now existing as H nuclei each with 4 constituent e-+/e+- particles) will combine, but they cannot do so within the boundaries of the proton that now have an excess number of e-+/e+- particles occupying available nuclear energy levels or shells. So one of the constituent e-+ particles moves way outside the proton boundary to occupy a distant energy level, or atomic orbital. This allows the new ‘orbital’ e-+ particle to interact with another ‘orbital’ e+- particle from a different proton that has also moved outside the boundary of its proton. This interaction forms a Hydrogen molecule.
Formation of Helium nuclei ….
If four ‘naked’ protons (each proton composed of four constituent e-+ and e+- particles) interact with each other simultaneously, they may ‘fuse’ to form a Helium atom. First, two of the ‘naked’ protons, each with four constituent e-+/e+- particles, ‘fuse’ to form a single nucleon that is equal to 2 X proton mass, now possessing eight constituent e-+/e+- particles – two more than a ‘normal’ nucleon equal to 2 X proton mass. Then, two of the ‘naked’ nucleons that equal 2 X proton mass interact with each other to ‘fuse’ into a single nuclear ‘boundary,’ resulting in a nucleus with two ‘naked’ nucleons entangled with each other, and occupying the same energy level within the newly formed nucleus. Each of the ‘naked’ nucleons equaling 2 X proton mass possess 8 constituent e-+/e+- particles, so that there is a total number of 16 constituent e-+/e+- particles in the newly formed He ‘naked’ nucleus – 4 more e-+/e+- particles than in a ‘normal’ He nucleus.
As the He ‘naked’ nuclei move away from the core of a star to regions of lower pressure and temperature, they are likely to experience instability. Since all the energy levels within the He ‘naked’ nucleus are filled beyond capacity (for lower pressure and temperature), the structure that was stable within the conditions of the core of a star no longer has optimal stability. So two of the four ‘excess’ constituent e-+/e+- particles move way outside the nucleus to a distant energy level (i.e., orbital) to provide greater directional balance and stability to the He nucleus. In the process, the other two ‘excess’ constituent e-+/e+- particles leave the He system entirely to become an entangled e-+ and e+- particle pair (i.e., analogous to an ‘electron pair,’ but with opposing e-m directionality).
[Coincidence?: The Compton (1-D wavelength) of the proton and its 3-D wavelength (E = 4πrk/r2; where k = energy at origin in a 3-D sphere) are the same – approximately 1320 millifermi (mfm). This allows exceptional structural stability of the proton(?).]
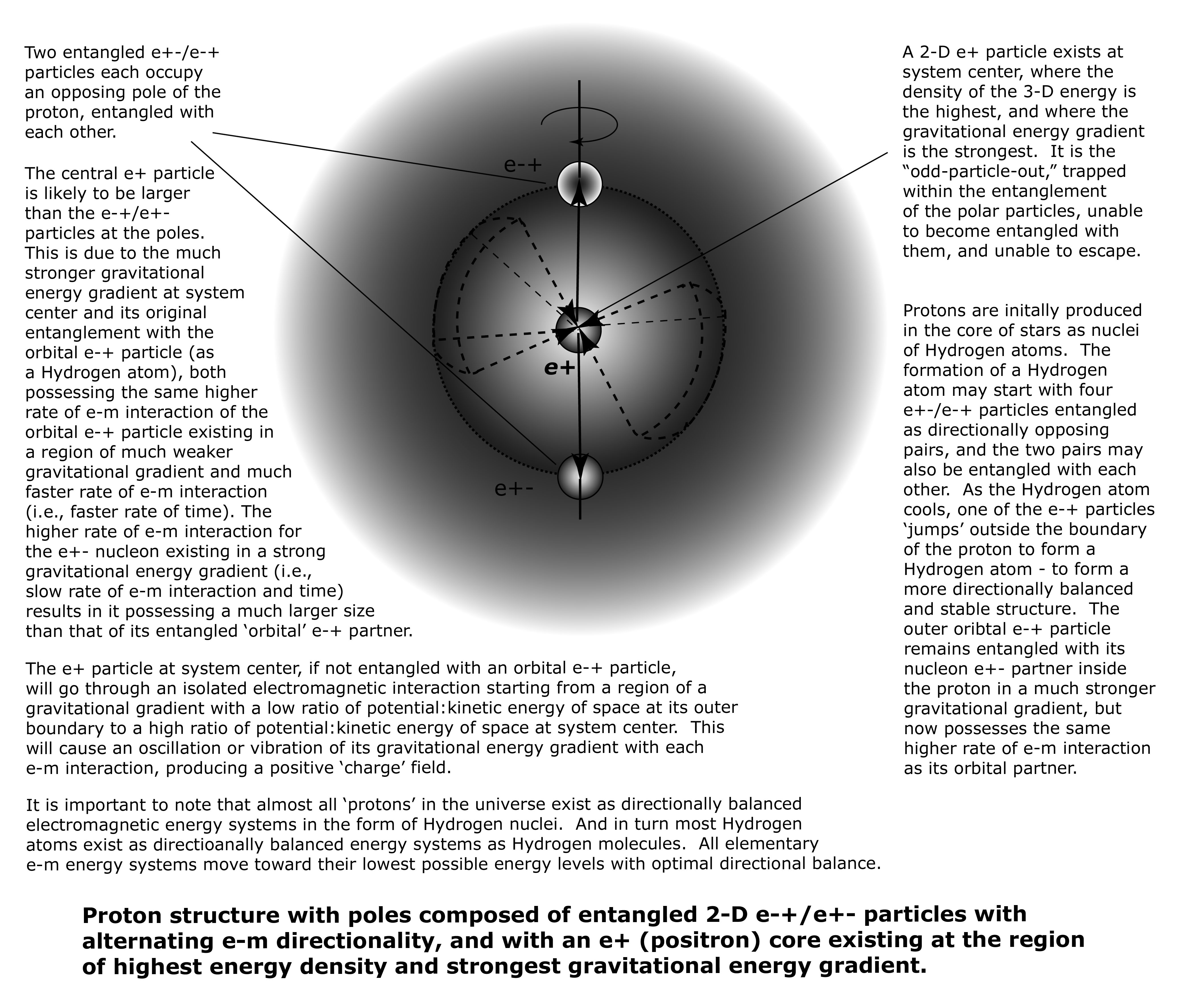
